This article is an excerpt from ESA SPCD 2022 paper entitled “Supercapacitors for space applications: trends and opportunities” written by Géraldine Palissat, Leo Farhat from ESA ESTEC and Joaquín José Jiménez Carreira, HE Space presented during the 4th ESA SPCD conference at ESA ESTEC, The Netherlands 11-14th October 2022. Published under ESA SPCD organisation committee permission.
ABSTRACT
Electric Double-Layer Capacitors (EDLC), also known as supercapacitors or ultracapacitors, are being considered as an energy storage option in space applications because they can operate over wider temperature ranges and have longer lifetimes and higher power densities than batteries. Since almost a decade, supercapacitors (SCs) were identified as promising high-power sources as they can bridge the gap between capacitors and batteries. SCs have been found to be potentially attractive for several space power applications. ESA has conducted several activities for developing supercapacitors for space applications. The trends and the opportunities of using supercapacitors in space applications will be discussed.
INTRODUCTION
The European Space agency (ESA) has been interested in the study of supercapacitors since the beginning of the 2000’s. Many activities have been released in order to study the benefits of supercapacitors in the energy storage systems of the spacecrafts and the launchers. At the beginning, the strategy was to identify the applications for which the use of supercapacitors could bring benefit compared to other electrochemical energy storage technologies, in terms of performances. Once the most relevant applications have been identified, the second step of the strategy was to qualify in space environment, supercapacitor devices used in terrestrial applications, also known as Commercial-of-the-shelf (COTS), that have demonstrated interesting performances for space applications. These COTS supercapacitors cells enabled to answer partially the high-power supply demands encountered in space applications as introduced in the following sections. Indeed, two factors enabled to explain that: the new requirements at supercapacitors part level in terms of power
and energy densities. Following the results obtained in the activity of space qualification of COTS supercapacitors, the third step of the strategy was to cover new requirements at supercapacitors devices level, with the main objective to design, develop and manufacture space qualified supercapacitors cells using innovative electrode materials that cope with the high-power demand not covered by the COTS supercapacitors nor by high power batteries and to replace obsolescent batteries technologies.
SUPERCAPACITORS FOR SPACE APPLICATIONS: MAIN TRENDS IN THE 2000’s
Since the early 2000’s, supercapacitors have been identified as interesting high-power sources. Indeed, supercapacitors have been identified as an adequate energy storage technology to ensure the peak power supply of several space applications ordered in function of their peak power requirements:
- Peaks power < 100W, like in robotic instrumentation, energy reserve for On-Board Computer, or Transmit and Receive Modules (TRM) for radar,
- Peaks power in the range of [100 W; 1 kW], as required for spacecraft actuator, pyrotechnics, radar and lidar, and launcher pyrotechnics and safeguard
- Peaks power > 10 kW, as needed for future radar, filtering bus for GEO spacecraft, and launchers Electromechanical Thrust Vector Control (EMTVC).
Several potential applications for telecommunications satellites, flight control and electric propulsion, have been identified in the past decades, with the main objective to use supercapacitors in order to optimise the overall mass and performances of the energy storage system, based on the promising peak power capabilities of these devices.
Some trends regarding each domain are listed below:
- Geostationary Earth Orbit subsystems: the objective is to use supercapacitors to keep a satellite’s power supply from fluctuating as the satellite loads change, providing Power Bus voltage regulation (start of electrical propulsion thrusters, eclipse transitions…). Another targeted application is the power supply of the release mechanisms of the spacecraft, used to deploy for example the solar panels, these are triggered after receiving a single pulse signal [3]
- High-power radar supply for small-satellite earth-observation missions ([3],[4]): the objective is to use supercapacitors to provide the necessary power and fulfil the low mass requirements [5]
- Flight control surface, including launch vehicle actuation systems [4]: the supercapacitors can provide the high-power density and energy storage that systems requires. A specific example of this hybridization is the Flight Control Actuation Systems (FCAS): these systems require high-power manoeuvres, while maintaining an adequate mission energy density. A USA patented Modular Electric Power Systems (MEPSTM), consisting of parallel strings of batteries supplemented with supercapacitors, complies with this requisite, saving weight and increasing the life of batteries at the same time
- Hybridization of a bank of supercapacitor (BOSC) with Li-Ion batteries for electrical Thrust Vector Control (TVC) in spacecrafts for collision avoidance purposes, ([3], [5]) and in launchers [6]: the objective is to use the supercapacitors to decrease the amount and duration of power loads on the batteries, and consequently extends the lifetime of the whole energy storage system [6]. In a formation flight frame, emergency collision avoidance within one hour is a necessity. Thrusters need a long pre-warming phase before any manoeuvre can take place
- Electric propulsion: a resistojet thruster that replaces the cold gas (Xe and Kr) system with supercapacitors managed to improve the specific impulse up to 28% [7], Optimization of the power supply of pyrotechnic separation mechanisms during launch phase ([4],[7], [8]): the objective is to replace the battery system by a bank of supercapacitors
- Mars exploration missions: ultra-low temperature supercapacitors (operating at – 70ºC) with porous carbon aerogel and advanced manufacturing technology have been identified as a potential power supply, in order to reduce the current heating needs for batteries [9]
If supercapacitors could be relevant, in order to power supply, the high-power peak demand of each of the applications listed above, their high-power capability is not enough to embed the technology is space applications. Indeed, there are a lot of other key parameters to consider for the optimization of the electrical architectures and the energy storage systems of spacecrafts and launchers.
SUPERCAPACITORS FOR SPACE APPLICATIONS: THE HIGH-POWER CHARACTERISTICS ARE NOT ENOUGH TO MAKE THE DIFFERENCE
The studies on supercapacitors, funded by ESA in the 2010’s have shown interesting results with regards to the capabilities and limitations of COTS supercapacitors. In one of these studies, entitled « high power battery-supercapacitor study » in 2010, carried out by EADS Space Transportation, the demonstration that the high power characteristics of COTS supercapacitors are not enough to make the difference in order to ensure the power supply of the various electrical functions of a spacecraft and of launcher with an hybrid power system composed of COTS supercapacitors and secondary batteries has been performed [10].
Indeed, there are several key parameters to consider:
- the range of energy, that is also quite broad depending on the targeted application, from few Joules for pyrotechnics function or Laser Imaging Detection and Ranging (LIDAR) to several hundred kilojoules for the power supply of large actuator such EMTVC for new launchers. So, it seems not feasible to cover such range of energy by a same high-power storage system. In some cases, as it is done in state-of-the art for energy storage solutions, the energy requirements could be covered with the parallelization of the high-power storage units. In such a case the knowledge of the failure modes of the unit is mandatory to cope with adequate “high power” system sizing.
- the range of operating voltage, with operating voltage requirements for spacecraft platforms from 28V up to 100V. For future launchers EMTVC is up to 400V. As it is observed in the state-of-the-art of the energy storage solutions, the series connection of high-power storage cells will be mandatory. Indeed, with respect to maximum operating voltage of such units of 4.10V for lithium-ion battery cells and of the order of magnitude of 2.85V for carbon/carbon supercapacitors, the design and the sizing of “high power” storage systems with several high-power cells in series shall be done in order to avoid stress on the single cells. It means that the overcharge or over-discharge of the single cells shall not occur or shall be limited during the mission life. Several solutions have been used in the state-of-the-art of energy storage solutions up to now: adequate matching of the single cells in series, use of passive or active “balancing system”, system oversizing.
- the range of temperature, with operating temperature requirements comprised in the range – 50°C and +70°C. As for any electrochemical power sources, the operational temperature has a strong influence on the electrochemical system performances.
- the mission duration range is between few hours (launchers) to several years with a maximum of around 20 years for telecom satellites (including 5 years storage), and an observed increase of mission duration for observation satellites up to 17 years (including 5 years storage). We can expect in the future the same trend with longer mission durations. Such mission durations mean high power storage sources must be compatible with such long mission duration. Such increase in mission durations will have an impact in term of qualification of this “high power storage sources”.
- And the number of cycles which covers a very broad range from some cycles to several millions cycling (bus filtering or actuator powering) depending on the targeted application. The cycling number will be one of key driver in the choice of the high-power storage power sources. For example, it is known that several millions cycling on a battery can be achieved only by using low Depth of discharge (DoD), meaning oversizing the electrochemical power system, and extra mass at system level. Supercapacitors are devices where the processes used to store and deliver the energy have been identified more suitable for very high number of cycles. Nevertheless, such device can compete to batteries only for very low energy requirements.
Finally, the results of the study have shown that the use of supercapacitors and/or of hybrid power sources instead of primary or secondary batteries could be particularly strategic for the following applications:
- spacecraft applications: high-power LIDAR, radars and high-power actuators. For each application, it has been demonstrated that the use of 10 F supercapacitor cells in hybrid power systems could be a decisive advantage by contributing to the limitation of the main bus perturbations due to peak power
- launcher applications: Electro-Mechanical Thrust Vector Control and pyrotechnics functions. Drastic gain in mass are expected by using 10 F to 100 F supercapacitor cells assembled in packs and used as single power source or combined with a battery pack to build a hybrid power system.
Indeed, the use of supercapacitors for space applications is still limited to some very specific applications. It’s mainly since in the meantime, the development and qualification of improved electrochemical storage devices such as lithium-ion batteries were achieved. The explanation for such differences in development between both technologies can be explained by the impact and the improvement of new technology on the spacecraft mainly in term of mass, cost, power, size, management for a given set of mission requirements: strong impacts and improvements were found for lithium batteries technologies and strong efforts were made by satellite equipment manufacturers to develop and qualify this technology.
Nevertheless, these main findings have paved the way to more than 10 years of research and development activities conducted by space industry in cooperation with supercapacitors manufacturers, under ESA funding.
SUPERCAPACITORS FOR SPACE APPLICATIONS: OPPORTUNITIES
These last years, with the growth market of the small spacecrafts, weighting between 100 kg and 200 kg, facing with constraints in the allowable on-board battery volume, new opportunities appear for the use of supercapacitors in space. Indeed, this volume constraint has an impact on the maximum power supply capability of small spacecrafts, which is generally limited in ranges between 70 W to 200 W. This relatively low maximum power limits the capabilities of small satellites in terms of payload design and selection. In order to enhance these satellites’ power performance, the implementation of supercapacitors as practical rechargeable energy storage medium, and as an alternative to chemical batteries is foreseen. Furthermore, as small spacecrafts programs are
deployed beyond Low-Earth Orbit (LEO), their energy storage system will have to operate at lower temperatures and under wider ranges of load capabilities.
The figure 1. hereafter presents the number of Small satellites by mass class, for the period 2012 – 2021.
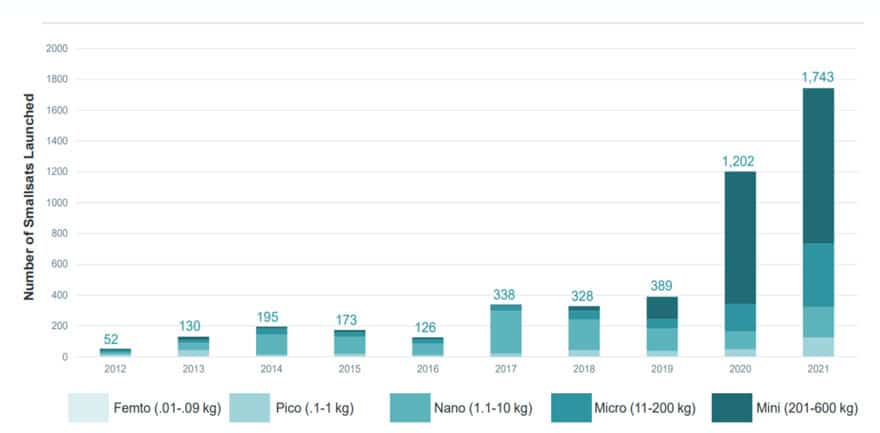
Many studies related to the use of supercapacitors in small satellites have been released these last years. The most interesting are listed hereafter:
- High power capabilities: researchers of the University of Surrey carried out a study in order to determine the feasibility and effectiveness of using supercapacitorsbased power systems. For that purpose, the basic operation methods and several topologies were proposed and examined through the simulation and analysed against surveys. In addition, a simple and easy to use formula for estimating discharge efficiency was derived. Both the simulations and the formula were validated by cross-checking. The results suggest that the supercapacitor can replace an on-board battery and provide high power capability to small satellites. However, current supercapacitor technology cannot practically store a whole orbit’s worth of average payload power at once, due to its low specific energy density. Current COTS very low ESR EDLCs have some potential to be used to supplement a battery for supplying very high power at high energy efficiency, for very short periods. However, LICs have advantages in terms of having higher energy capacity. indeed, the provision of this high-power capability would considerably widen the range of small satellite applications, enabling to reach 1 kW peak power capabilities and overpass the 200W peak power limitation [5].
- Hybrid power supply: in a NASA funded work, carried out at the Jet Propulsion Laboratory in 2013, a hybrid energy storage system consisting of a low temperature Li-ion cell (26 650 Li-ion battery cell – 2.3 Ah) and a bank of supercapacitors (310F Maxwell Technologies Inc.) was evaluated for performances enhancements at high power and low-temperature for future deep-space CubeSat applications (CSUN Cubesat). Although no significant improvements are observed in terms of the energy storage as compared to a CubeSat polymer battery, the hybrid power system exhibited substantial gains in power with high current (15A) pulse testing. The minimum discharger voltage is about 2.0V higher than the standalone Li-ion cell at the worst case initial 50% State-of-Charge. Finally, another benefit, is the low impedance of the hybrid system which is responsible for the observed performance improvement is about 5mOhm at a -40 oC operating temperature and is substantially lower than the CubeSat baseline polymer battery of about 1000 mOhm [15].
- 1U CubeSat Electrical Power System: a new EDLC-based EPS board was developed and tested in this study for its electrical performance and robustness in space environments, including launch environments and thermal environments. The total EDLC capacitance was 1600 F, the Electrical Power System occupying a volume of 90 × 87.3 × 64.6 mm. The functionality of the board was tested, assuming realistic power, voltage, and current profiles based on actual orbital periods, and assuming release of the satellite from the International Space Station (ISS). The CubeSat power consumption profile was assumed to be from 920 mW to 2.67 W, and the photovoltaic power generation output to be 2.93 W, at its peak. The board was proven to withstand space environments, and to provide the power to operate a CubeSat in orbit, with a remaining energy level of 52% at the end of eclipse [16].
- In-orbit demonstration of COTS supercapacitors: in this study, a commercially available (COTS) supercapacitor (400 F supercapacitor cell manufactured by PowerStor/Eaton, XV series) was selected to power supply one of the payloads of the spacecraft Ten-Koh and wasn’t used as an energy storage medium for the spacecraft electrical power system. Indeed, the supercapacitor was considered a part of the spacecraft payload and, because it did not serve as primary energy storage for the spacecraft, any potential failure was considered acceptable to the mission as whole. Ten-Koh was launched on October 2018 and remained operational until mid March 2019. To contribute to studies that support the feasibility of using supercapacitors in small spacecraft, two goals are pursued: First, to demonstrate that a supercapacitor can survive the launch. Second, to prove that charging and discharging a supercapacitor in Low Earth Orbit (LEO) is possible. Three charge discharge cycles of the supercapacitor in LEO have been recorded over the timespan of five months from the launch and are compared to ground results. In addition, selfdischarge over two days in orbit has been calculated as less than 2.5%. These results show that the COTS supercapacitor can withstand the launch and space environment with capacitance loss lower than 1% and, therefore, is a viable energy storage device for LEO satellites. However, degradation of the supercapacitor over longer stays in orbit and repeated cycling is yet to be characterised [17].
CONCLUSIONS
The studies reported in this paper have demonstrated the successful use of supercapacitors, in the power supply and/or the hybrid power supply of several classical spacecrafts’ applications (high-power LIDAR, radars and high-power actuators) and of two functions of launchers (pyrotechnics and EMTVC).
Furthermore, the studies have shown the limitations and constraints related to the use of COTS supercapacitors. Indeed, enhancement of the performances of existing supercapacitors is an innovative field where new materials (VACNTs, graphene…) are going to play a determinant role in order to develop high-energy supercapacitors (> 15 Wh/kg) and hybrid supercapacitors (e.g Lithium Carbon capacitors with 50 Wh/kg energy density).
With the proliferation of small satellites in recent years, the use of COTS supercapacitors that have not been developed for space applications is of interest to the space community. The use of COTS supercapacitors is interesting in more than one way as shown in the paper.
Indeed, COTS supercapacitors have demonstrated their capability to be used: as the electrical power system of 1U CubeSat, as a payload power supply, as an electrical performances enhancer in a hybrid-power supply of small spacecraft.
Finally, innovative supercapacitors based on graphene could be an enabler for the small satellites market, enabling to propose improved and/or new services and extended life duration.
REFERENCES
[1]: F. Beguin, E. Frackowiak, Ed., Supercapacitors: Materials, Systems and Applications (Wiley-VCH, Weinheim, Germany), 2013.
[2]: L.H. Heß, S. Wankmüller, A. Bothe, A. Balducci, Safe, low-cost and SEI forming electrolytes for lithium-ion batteries based on glyoxylic acetal solvents, Batteries & Supercaps, 2, 852– 857, 2019.
[3]: Graphene Enabled Supercapacitors Cell, ESA-GRACE-FR, Pleione Energy, 2010.
[4]: S. Steffan, G. Semrau, High power density modular electric power system for aerospace applications, Moog Inc, Space and Defence Group, New York (USA), 2013.
[5]: T. Shimizu, C. Underwood, Supercapacitor energy storage for micro-satellites, Surrey Space Centre, University of Surrey, UK, Acta Astronautica 85, 138-154, 2014.
[6]: M. Zimmermann, C. Buffry, SafeCap: Ionic liquids Supercapacitor, Hutchinson Research Centre, Chalette-Sur-Loing, France, 2013.
[7]: J.Kindracki, P. Paszkiewicz, Ł. Mężyk, Resistojet thruster with supercapacitor power source – design and experimental research, Aerospace Science and Technology 92, 847-857, 2019.
[8]: B. Faure, L. Cosqueric, V. Gineste, D. Latif, P. Vasina, D. Lacombe, B. Bürgler, M.Simcak, Evaluation and qualification of commercial off-the-shelf supercapacitors for space applications, 2nd Space Passive Component Days (SPCD), 2018.
[9]: B. Yao, H. Peng, H. Zhang, J. Kang, C. Zhu, G. Delgado, D. Byrne, S. Faulkner, M. Freyman, X. Lu, M. A. Worsley, J. Q. Lu, and Y, Li, Printing Porous Carbon Aerogels for Low Temperature Supercapacitors, University of California, USA, Nano Lett. 2021, 21, 9, 3731–3737, 2021.
[10]: P. Mattesco, B. Kieve, N. Neugnot, J. Labbe, J-P LE ROY, P. Simon, High power battery supercapacitor study final report, 2010.
[11]: L. Farhat, J. Jimenez Carreira, G.Palissat, Supercapacitors: Applications in Space, Development Conducted by ESA and Challenges to Overcome, 2021.
[12]: M. Arulepp, J. Leis, High Power and Energy Density Ultracapacitors in space environment, Mati Arulepp, Jaan Leis, Skeleton Technologies, Estonia; SpaceCap and SpaceCap modules Final Report.
[13]: M. Lichtenberger, A. Boisset, G. Palissat, Supercapacitor for launcher applications, Final Report, ALMATECH (Switzerland), NawaTechnologies (France), Ariane Group (France), 2018.
[14]: Smallsats by the Numbers, BRYCE Tech, 2022.
[15]: K.B. Chin, M.C. Smart, E.J. Brandon, G.S. Bolotin, N.K. Palmer, Lithium-ion and supercapacitor hybrid energy storage system for low temperature smallsat applications, 28th Annual AIAA/USU Conference on small satellites, 2014.
[16]: Kim, S. et al., Design, Fabrication, and Testing of an Electrical Double-Layer Capacitor-Based 1U CubeSat Electrical Power System Journal of Small Satellites, Vol. 7, No. 1, pp. 701–717, 2018.
[17]: J. Gonzalez-Llorente, A. A. Lidtke, K. Hatanaka, L. Limam, I. Fajardo, K.I. Okuyama, In-orbit feasibility demonstration of supercapacitors for space applications, Acta Astronautica 174, 294-305, 2020