This article presents a two-step process that involves pyrolysis followed by chemical activation, which effectively transforms common plastic waste into activated carbons (ACs), making them suitable for electrode materials in supercapacitors.
The paper was presented by Ivan Dฤdek, Czech Advanced Technology and Research Institute (CATRIN), Palackรฝ University, Olomouc, Czech Republic at the 4thย PCNSย 10-14thย September 2023, Sรธnderborg, Denmark as paper No. 2.7.
Abstract
Activated carbons (ACs) have common application for the EDLC (electric double layer capacitance) supercapacitors. Among various methods, ACs can be manufactured from the plastics, which offers a sustainable method for managing plastic waste. In this study, we present a two-step process that involves pyrolysis followed by chemical activation, which effectively transforms common plastic waste into activated carbons (ACs), making them suitable for electrode materials in supercapacitors.
In addition to well established parameters such as specific surface area and micropore volume, our research highlights the importance of other critical factors, including the glass transition temperature of the polymer, compatibility between the polymer and activating agent, the ratio of activating agent (K2CO3) to ACs, and the stability of ACs in water dispersion. Tuning the mentioned parameters, we obtained AC with a competitive electrochemical performance. Specifically, the ACs exhibited a specific capacitance of 220 F gโ1 (at a current density of 1ย Aย gโ1), energy and power densities of 61.1 Wh kgโ1 and 36.9 kW kgโ1, respectively, and excellent cycling stability (95% retention after 30,000 cycles). Our findings provide a pathway towards transforming plastic waste into valuable electrode materials for supercapacitors.
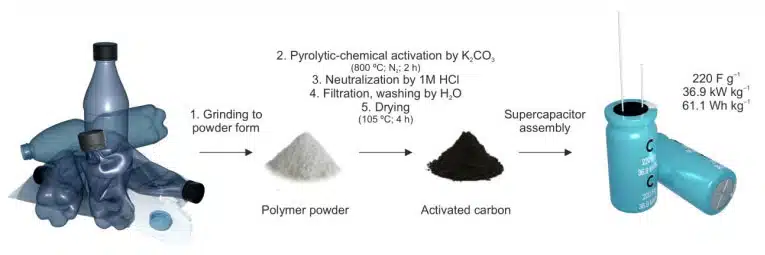
Introduction
Environmental pollution on a broad scale is caused by the pervasive plastic waste. In order to avoid its combustion and landfilling, it is required to look into various techniques for its recycling or transformation. According to global estimates, the amount of plastic waste generated each year is approximately 3ร108 tons.1 While recycling is considered a way to utilize plastic waste, only 9% of plastic waste is recycled worldwide, with 20% being mismanaged.2 The remaining 60-70% of plastic waste is largely burned or disposed of in landfills. Rather than resorting to incineration, an alternative approach is to transform plastics into valuable materials, such as activated carbons (ACs), which offers a sustainable method for managing plastic waste.
Supercapacitors, can be utilized to provide high power output, reduce battery discharge depth, and store energy for memory operations in case of unexpected shutdowns. Compared to batteries, supercapacitors have the advantage of fast charging and discharging, making them optimal for energy harvesting and scavenging. Depending on power requirements, supercapacitors can even replace batteries entirely. It is simple to understand why capacitor technology is the best choice for energy storage applications when you consider the better power density of supercapacitors along with its wide working temperature range, excellent reliability, low weight, and high efficiency.
The mechanism of EDLC SCs is simply based on electrostatic adsorption of ions, where the suitable pore size (preferably micropores3) with the high SSA plays a main role in the high energy storage performance. As a result, such SCs display enormous cycling stability, fast charging and discharging ability, and enhanced power density.
In this contribution is discussed the conversion process of common plastic waste materials, including polyethylene (PE), polypropylene (PP), polystyrene (PS), polyamide (PA), polyester fabric (PES), polyethylene terephthalate (PET), polyurethane (PU), and acrylonitrile-butadiene-styrene (ABS), into activated carbons (ACs) that possess appropriate electrochemical characteristics for supercapacitor applications. Our findings indicate that, in addition to the specific surface area (SSA) and porosity of the ACs, several other factors significantly influence the capacitive properties of ACs. These factors include the glass transition temperature (Tg) of the polymer, the compatibility of the polymer with K2CO3 as an activating agent, and the stability of AC water dispersion.
Polymer Transformation
We describe the transformation of common plastic waste materials, such as polyethylene (PE), polypropylene (PP), polystyrene (PS), polyamide (PA), polyester fabric (PES), polyethylene terephthalate (PET), polyurethane (PU) and acrylonitrile-butadiene-styrene (ABS), into ACs with suitable electrochemical parameters towards supercapacitor applications.
PS and PET pellets were easily processed without any manufacturing difficulties, while polymers like PE, PP, PU, and ABS posed challenges during milling due to sintering. Sintering occurred because these polymers reached their glass transition temperature (Tg), causing their structure to become rubbery and complicating the grinding process. The sintering also hindered the mixing of K2CO3, leading to decreased specific capacitance of the activated material. This was evident from the specific capacitance values obtained for each polymer (Fig. 1 a).
We also observed visible differences in the dispersibility of the AC materials in water. Some of the materials formed agglomerates and quickly settled down, while others formed stable dispersions. These variations could be attributed to differences in surface energy among the AC materials.The C-PS sample, which had a specific surface area (SSA) of 750 m2 gโ1 did not form agglomerates, exhibited capacitance values similar to C-PU, which had an SSA of 1559 m2 gโ1 and formed agglomerated dispersions. Additionally, C-PES, with an SSA of 909 m2 gโ1, formed very fine dispersions. From these observations, we concluded that the combination of high surface area, high micropore volume, and the ability to form fine dispersions were responsible for the observed capacitance.
The sample exhibiting the highest capacitance value (C-PA) was chosen to investigate the impact of the AC: K2CO3 ratio on capacitance properties (Fig. 1 b). As the amount of K2CO3 decreased, the specific surface area (SSA) increased up to a C-PA: K2CO3 ratio of 1:0.5, reaching a remarkably high SSA of 2266 m2 gโ1. However, this trend did not correspond to an increase in capacitance. Carbon with a lower K2CO3 ratio was found to be ineffective in terms of capacitance due to its poor ability to form stable dispersions. The AC material exhibited instability and quickly aggregated. This behaviour resulted in low-quality films on the electrode surface, leading to lower capacitance values.
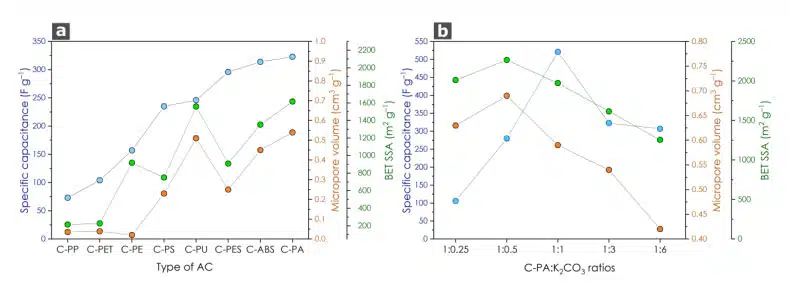
Although the samples with C-PA: K2CO3 ratios of 1:3 and 1:6 had lower SSA values compared to the others, they formed stable dispersions and still exhibited high capacitance values of 350ย F gโ1 and 300 F gโ1, respectively. The peak performance of 521 F gโ1 (at 1 A gโ1) was achieved when the C-PA: K2CO3 ratio was 1:1. Deviating from this ratio, whether by increasing or decreasing it, led to a deterioration in sample performance, as evidenced by the GCD test (Fig. 2 d, e). This can be attributed to the ability to form stable dispersions. Samples with higher capacitance values tend to form stable dispersions, which have been proven to be highly beneficial from an electrochemical perspective. In contrast, the C-PA samples, despite having a high SSA, form unstable dispersions and quickly aggregate. This results in significantly lower capacitance values from an electrochemical standpoint.
The AC samples derived from C-PA, C-ABS, C-PES, and C-PU that exhibited the highest performance were subjected to further characterization using a 2-electrode symmetrical setup, which closely resembles a real supercapacitor device (Fig. 2). The highest specific capacitance of the C-PA among all the carbon samples is attributed not only to the very high specific surface area and the micro-pore volume, but also to the ability to form stable dispersion, which eventually benefits the adsorption of the higher amount of the electrolyte ions. These micropores responsible for the high amount of the accommodated electrolyte ions and the immensely high capacitance. C-PA 1:1 exhibited a micropore: total pore volume ratio 63ย %, which predisposes it for the fabrication of the EDLC supercapacitor. C-PA 1:1 with best capacitance results (521ย Fย gโ1) had the specific surface area from BET equaled to 1974ย m2ย gโ1 with a total micropore volume of 0.59 cm3 gโ1.
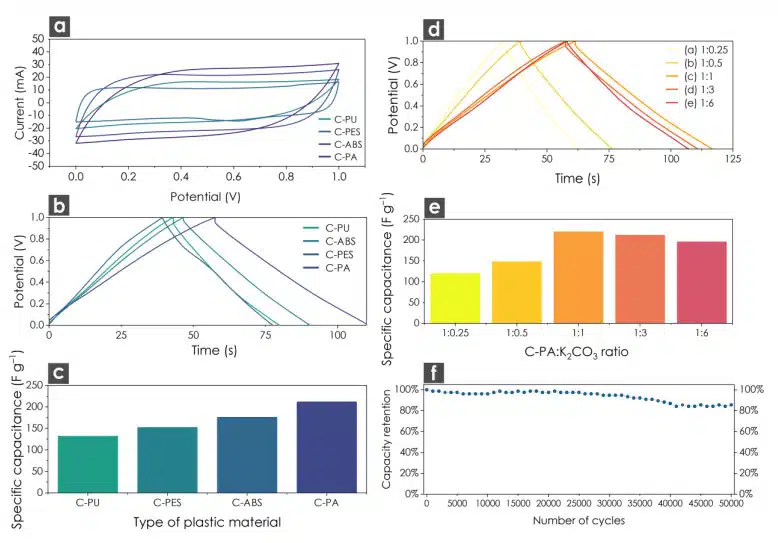
In order to explore the applicability of the C-PA sample, we utilized an ionic liquid, [EMIM][BF4], as an electrolyte to enhance the capacitive performance in terms of energy and power density. Ionic liquids are a type of electrolyte commonly employed to achieve higher capacitance and energy density. We specifically selected 1-ethyl-3-methylimidazolium tetrafluoroborate ([EMIM][BF4]) as the electrolyte due to its ability to operate within a wide potential window and its molecular size matching the pore width of our ACs.4 The stable potential window was investigated using cyclic voltammetry (CV) in the range of 0-3.7 V. The CV curves displayed a semi-rectangular shape without observable peaks. The galvanostatic charge-discharge (GCD) measurements at various current densities exhibited high energy density and power density, as illustrated in Figure 3a. The Ragone plot (Figure 3b) compares our findings with relevant publications on ACs derived from plastic waste. In our study, we achieved a maximum energy density of 61.1 Wh kgโ1 and a maximum power density of 36.9 kW kgโ1. These results significantly surpass previously published outcomes, suggesting that the C-PA material could serve as a promising alternative to commercially available carbons.
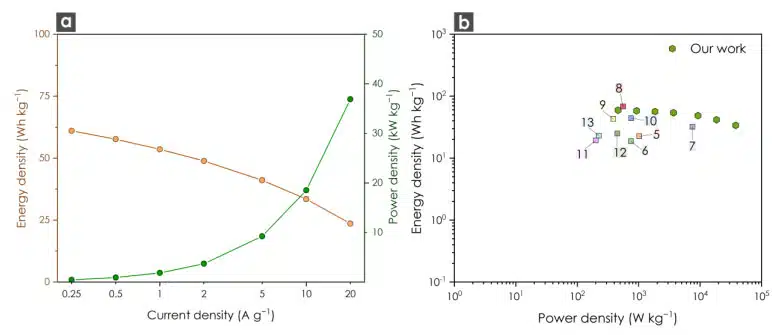
Conclusion
To meet the demand for sustainable electronics and plastic waste reuse, we successfully obtained highly porous activated carbon (AC) materials from common plastic waste for supercapacitor electrodes.
The final supercapacitive properties are influenced by several key parameters, including not only well-known specific surface area (SSA) and microporosity but also the glass transition temperature of the polymer, compatibility between the polymer and activating agent (K2CO3), and the stability of the activated carbon (AC) dispersion. The identification of these critical parameters, together with the outstanding results obtained, represents a significant advancement towards the efficient utilization of plastic waste in energy storage devices.
The C-PA sample, exhibiting the best properties, achieved a capacitance of 220 F gโ1 (at 1 A gโ1) and excellent cyclic stability (95% retention after 30,000 cycles) in an aqueous electrolyte. In an ionic liquid electrolyte, the C-PA sample showed significantly improved energy and power density (61.1 Wh kgโ1 and 36.9 kW kgโ1).
Our findings emphasize the importance of multiple parameters in obtaining high-performance electrode materials, such as surface area, micropore volume, dispersion quality, pore size distribution, K2CO3:AC ratio, and precursor’s glass transition temperature. Careful evaluation of these factors allowed us to develop novel sustainable materials with superior electrochemical properties in terms of both capacitance and stability for supercapacitor applications.
References
(1) Ritchie, H.; Roser, M. Plastic Pollution. Our World in Data 2018.
(2) OECD. Global Plastics Outlook: Economic Drivers, Environmental Impacts and Policy Options; Organisation for Economic Co-operation and Development: Paris, 2022.
(3) Chmiola, J.; Yushin, G.; Gogotsi, Y.; Portet, C.; Simon, P.; Taberna, P. L. Anomalous Increase in Carbon Capacitance at Pore Sizes Less Than 1 Nanometer. Science 2006, 313 (5794), 1760โ1763. https://doi.org/10.1126/science.1132195.
(4) Sun, L.; Zhuo, K.; Chen, Y.; Du, Q.; Zhang, S.; Wang, J. Ionic Liquid-Based Redox Active Electrolytes for Supercapacitors. Advanced Functional Materials 2022, 32 (27), 2203611. https://doi.org/10.1002/adfm.202203611.
(5) Zhang, Y.; Shen, Z.; Yu, Y.; Liu, L.; Wang, G.; Chen, A. Porous Carbon Derived from Waste Polystyrene Foam for Supercapacitor. J Mater Sci 2018, 53 (17), 12115โ12122. https://doi.org/10.1007/s10853-018-2513-z.
(6) Lian, Y.-M.; Utetiwabo, W.; Zhou, Y.; Huang, Z.-H.; Zhou, L.; Faheem, M.; Chen, R.-J.; Yang, W. From Upcycled Waste Polyethylene Plastic to Graphene/Mesoporous Carbon for High-Voltage Supercapacitors. Journal of Colloid and Interface Science 2019, 557, 55โ64. https://doi.org/10.1016/j.jcis.2019.09.003.
(7) Chodankar, N. R.; Patil, S. J.; Hwang, S.-K.; Shinde, P. A.; Karekar, S. V.; Raju, G. S. R.; Ranjith, K. S.; Olabi, A. G.; Dubal, D. P.; Huh, Y. S.; Han, Y.-K. Refurbished Carbon Materials from Waste Supercapacitors as Industrial-Grade Electrodes: Empowering Electronic Waste. Energy Storage Materials 2022, 49, 564โ574. https://doi.org/10.1016/j.ensm.2022.04.039.
(8) Elessawy, N. A.; El Nady, J.; Wazeer, W.; Kashyout, A. B. Development of High-Performance Supercapacitor Based on a Novel Controllable Green Synthesis for 3D Nitrogen Doped Graphene. Sci Rep 2019, 9 (1), 1129. https://doi.org/10.1038/s41598-018-37369-x.
(9) Lian, Y.; Ni, M.; Huang, Z.; Chen, R.; Zhou, L.; Utetiwabo, W.; Yang, W. Polyethylene Waste Carbons with a Mesoporous Network towards Highly Efficient Supercapacitors. Chemical Engineering Journal 2019, 366, 313โ320. https://doi.org/10.1016/j.cej.2019.02.063.
(10) Ma, C.; Liu, X.; Min, J.; Li, J.; Gong, J.; Wen, X.; Chen, X.; Tang, T.; Mijowska, E. Sustainable Recycling of Waste Polystyrene into Hierarchical Porous Carbon Nanosheets with Potential Applications in Supercapacitors. Nanotechnology 2020, 31 (3), 035402. https://doi.org/10.1088/1361-6528/ab475f.
(11) Ma, C.; Min, J.; Gong, J.; Liu, X.; Mu, X.; Chen, X.; Tang, T. Transforming Polystyrene Waste into 3D Hierarchically Porous Carbon for High-Performance Supercapacitors. Chemosphere 2020, 253, 126755. https://doi.org/10.1016/j.chemosphere.2020.126755.
(12) Liu, X.; Wen, Y.; Chen, X.; Tang, T.; Mijowska, E. Co-Etching Effect to Convert Waste Polyethylene Terephthalate into Hierarchical Porous Carbon toward Excellent Capacitive Energy Storage. Science of The Total Environment 2020, 723, 138055. https://doi.org/10.1016/j.scitotenv.2020.138055.
(13) Liu, X.; Ma, C.; Wen, Y.; Chen, X.; Zhao, X.; Tang, T.; Holze, R.; Mijowska, E. Highly Efficient Conversion of Waste Plastic into Thin Carbon Nanosheets for Superior Capacitive Energy Storage. Carbon 2021, 171, 819โ828. https://doi.org/10.1016/j.carbon.2020.09.057.